Updated on June 2, 2019
Chiral Plasmonic Nanomaterials Prepared by Circularly Polarized Light
We synthesized chiral plasmonic nanomaterials by circularly polarized light for the first time. Chirality was given to those nanomaterials by twisting their structures clockwise or anticlockwise by plasmon-induced charge separation (PICS) under circularly polarized light. Potential applications of the nanomaterials include nanoscale light sources for circularly polarized light, chirality sensors, chiral photocatalysts, and metamaterials. [Nano Lett, 2018]
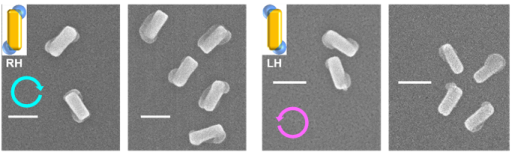
Plasmon-Induced Charge Separation with a Compound Nanomaterial
Since we found plasmon-induced charge separation (PICS), it has been based exclusively on noble metal nanomaterials. Recently we achieved PICS with a plasmonic compound nanomaterial for the first time. Its applications include near infrared photodetection. [Nanoscale, 2018]
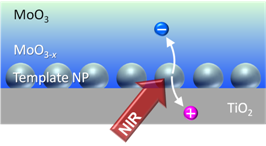
Construction of Nanocities
The cuboidal objects that look like buildings in the electron micrograph are, actually, silver nanoparticles. The nanocity was constructed on a TiO2 substrate on the basis of plasmon-induced charge separation (PICS). Each nanoparticle exhibits at least three different plasmon modes, so that it would be used for data storage. [Nanoscale, 2017]
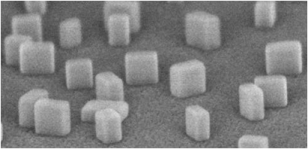
A New Synthetic Method for Plasmonic Molybdenum Oxide Nanoparticles
We developed a new method for synthesis of MoO2 and MoO3-x nanoparticles in non-aqueous media. Oxidative conversion of MoO2 nanoparticles to MoO3-x nanoparticles was also achieved. [Chem Commun, 2017]
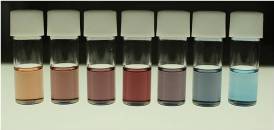
Semi-Transparent Solar Cells Developed by Considering Human Visual Sensitivity
If you make a solar cell more transparent, it will use less photons and you will lose power. We addressed this trade-off, keeping in mind that human eyes are not so sensitive to blue and red light. We coupled perovskite solar cells, which are sensitive to blue light, with plasmonic silver nanocubes, which enhance red light absorption of the perovskite material. As a result, the plasmonic perovskite solar cells achieved energy conversion efficiency of about 10% despite good visual transparency. [Sci Rep, 2017]
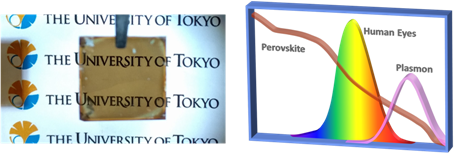
Single Plasmonic Nanoparticles for Chemical Sensing
We have developed potential-scanning LSPR sensors, which need no spectrophotometer in 2015. We worked further on it with Prof. Mulvaney and his co-workers and used single gold nanorods and nanorod dimers as single channels of a multiple potential-scanning LSPR sensor. [JPCL, 2017]
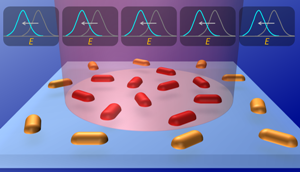
Decomposition of Odorous Organic Species after Dark
We have designed various types of photocatalysts with energy storage abilities. Here we report that odorous multicarbon compounds including acetaldehyde, acetic acid, and acetone can be decomposed to CO2 by an oxidative ability of the energy-stored photocatalysts. [PCCP, 2016]
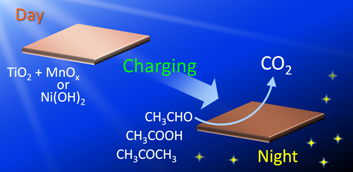
Photochemical Partial Etching of Nanoparticles Smaller than Light Wavelength
We developed a technique for selective etching of the top or bottom of silver nanocubes on the basis of plasmon-induced charge separation (PICS). It would be applied to high-density data storage. More importantly, this effect is the first experimental evidence of hole ejection involved in PICS. [JPCL, 2016]

Translucent Films Showing Different Colors on Each Side [Movies!]
By taking advantage of strong light scattering from plasmonic metal nanoparticles, we developed translucent films that show different colors on each side. Two different types of plasmonic nanoparticles are embedded at different depths in a film, so that one of them is excited by the front side irradiation, and the other one is excited by the back side irradiation. This class of materials would be applied to security printing and advanced coloring. [ACS Photonics, 2016]
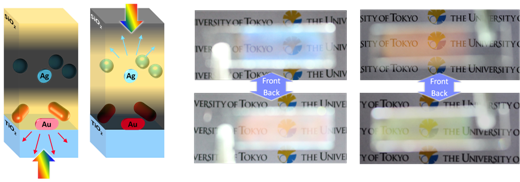
Anodic Potential of Plasmon-Induced Charge Separation
We investigated anodic potentials of plasmon-induced charge separation (PICS) on the basis of hydroxylation of gold nanoparticles, and found that the potential can be controlled by changing solution pH and light wavelength. [Angew Chem Int Ed, 2016]
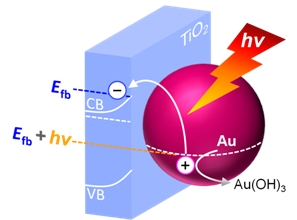
Near Infrared Smart Windows
Not only noble metal but also some compound nanoparticles exhibit plasmon resonance. We developed near infrared smart windows, whose ability for near infrared light absorption based on plasmon resonance can be switched off and on, by reducing and oxidizing CuS nanoparticles, respectively, by electrochemical means. [Nanoscale, 2016]
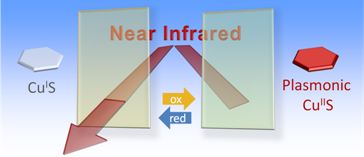
Transparent Projection Screen
We developed a transparent film, on which images can be projected. The screen is based on submonolayer of silver nanocubes whose scattering band is broadened by a highly refractive film. [Nanoscale, 2015]
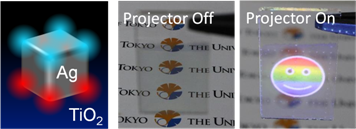
LSPR Sensors without Spectrophotometer
Conventional LSPR sensors require a spectrophotometer, which scans light wavelength and detects LSPR peak shifts of plasmonic nanoparticles. Spectrophotometers are large and expensive in general, and small ones lack accuracy. So we immobilized nanoparticles onto a transparent electrode and scanned its potential under monochromatic light, instead of scanning the wavelength. The sensor no longer requires a spectrophotometer, so that it would be smaller and less expensive. [ACS Nano, 2015]
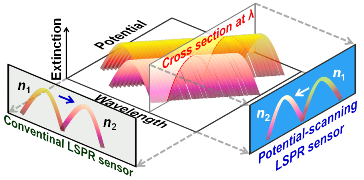
Asymmetric Color Routers [Movie!]
We developed an asymmetric color router, which looks almost transparent and colorless under ambient light. Under direct irradiation, however, it shows blue scattered, yellow reflected, and red transmitted lights in the case of front incidence. It shows yellow scattered, blue reflected, and red transmitted lights for back incidence. The asymmetric behavior is based on plasmon resonance of silver nanocubes on titania. The colors can be changed by tuning the titania thickness and cube size. [Adv Opt Mater, 2015]
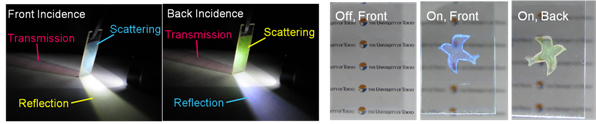
Efficiency Enhancement of Colloidal Quantum Dot Solar Cells by Plasmonic Nnocubes
We worked with Prof. Segawa, Prof. Kubo, and their co-workers for efficiency enhancement of solar cells. Power conversion efficiency of bulk-heterojunction solar cells with colloidal PbS quantum dots and ZnO nanowires was improved from 4.5% to 6.0% by loading with plasmonic silver nanocubes. [ACS Nano, 2015]
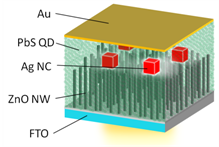
LSPR Sensors for Colored or Turbid Samples
SPR sensors based on gold films are widely used for affinity-based chemical sensing and biosensing. LSPR sensors based on plasmonic nanoparticles also attract attention because they can be smaller and less expensive. However, in general, LSPR sensors are not applicable to colored or turbid sample solutions because light must pass through the sample to an optical detector. We developed LSPR sensors that output electrical signals (potential or conductivity changes) directly, on the basis of plasmon-induced charge separation (PICS). The sensors do not require light to pass through the sample because of the direct output. We demonstrated that the sensors can be applied to coffee as a colored and turbid sample. [Chem Commun, 2015]
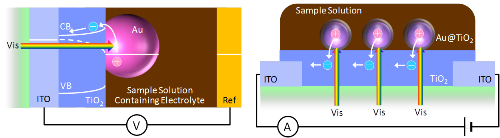
Solid-State Photovoltaic Cells Based on Metal Clusters
We have proposed and developed metal cluster-sensitized photovoltaic cells (or metal cluster-sensitized solar cells) for the first time in 2010. However, the cells required a liquid electrolyte. So we developed metal cluster/semiconductor heterojunction cells by using Au25 clusters and TiO2. [Appl Phys Lett, 2014]
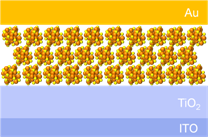
Nanoscale Photopotential Measurements for Plasmon-Induced Charge Separation
We have found plasmon-induced electron transfer from plasmonic nanoparticles to semiconductor for the first time. It is "plasmon-induced charge separation (PICS)", which is recently also known as "plasmon-induced hot electron injection", and is now studied by many other research groups in the world. We observed bidirectional photoinduced electron transfer for triangular gold nanoplates deposited on TiO2 by means of Kelvin probe force microscopy (KFM). Electrons are transferred from TiO2 to gold when TiO2 is excited by UV, and from gold to TiO2 when the nanoplates are excited by near IR. [Adv Mater Interfaces, 2014; featured on the backcover]
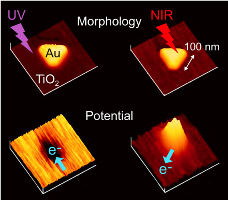
Wavelength-Tunable LSPR Sensors
Plasmonic nanoparticles are sensitive to local refractive index changes and their absorption peak redshifts in response to an increase in the refractive index. So the nanoparticles are used for LSPR sensors, which can be applied to chemical sensors and biosensors including immunosensors. The absorption peak should appear in a spectral window, in which the sample solution has no excess absorption. We developed a new class of LSPR sensors based on "absorption dips" that can be formed at a desired wavelength simply by intense irradiation at the corresponding wavelength prior to the measurements. [Nanoscale, 2014]
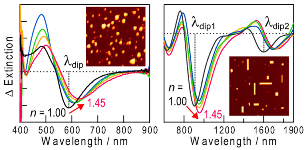
Gold Cluster-Nanoparticle Diads
We have proposed and developed metal cluster-sensitized photovoltaic cells, and their internal quantum efficiency (IQE or APCE) is up to 80%. On the other hand, plasmonic nanoparticles are characterized by high photoabsorption. So we developed the gold cluster-nanoparticle diad system to combine those two advantages. As a result, the photosensitization was enhanced by 9-fold in the near infrared region. [Nanoscale, 2013]
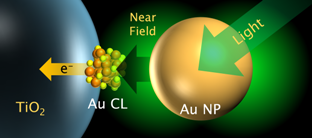
Photocurrent Enhancement in Near IR by Plasmon Coupling
Photocurrents of solar cells are known to be enhanced by plasmonic nano-antennae. The enhancement was improved further in the near infrared region by taking advantage of a plasmon coupling effect, which gives far stronger electric fields in the small gap between the neighboring nanoparticles. [J Phys Chem C, 2013; featured on the cover]
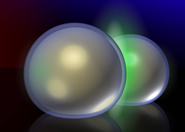
Multicolor Changes with Gold Nanoparticles
We previously reported multicolor photochromism based on spectral dip formation of plasmonic silver nanoparticles. Now it is also possible with gold nanoparticles by the aid of "ligands". The formed spectral dips are more stable than those for silver nanoparticles. [Chem Commun, 2013]

Multicolor Changes with Single Silver Nanoparticles
Photoinduced multicolor changes are now possible with single silver nanoparticles. We place a simple spherical silver nanoparticle on TiO2 with high refractive index and separate a plasmon mode localized at the Ag-TiO2 interface from the other mode distributed over the nanoparticle. Selective excitation of each mode gave corresponding morphological changes and resulted in selective suppression of the plasmon mode and multicolor changes. Potential applications include nanophotonic devices. [Nano Lett, 2012]

Charge Separation Induced by Dark Plasmons
So far, plasmon-induced charge separation (PICS) has been based on dipole (bright mode) plasmons. Here we found PICS based on multipole (dark mode) plasmons. Therefore, long nanorods whose dipole resonant energy is lower than the energy threshold for PICS can also be used for PICS. [J Phys Chem C, 2012 (Invited Paper)]
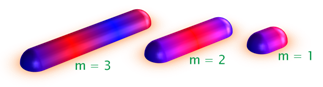
Plasmon-Induced Charge Separation with Copper Nanoparticles
We have found plasmon-induced charge separation (PICS) at the interface between a semiconductor such as TiO2 and a plasmonic nanoparticle of silver or gold. Now PICS is also possible with copper nanoparticles, which are more cost-effective than silver and gold. [Chem Lett, 2012 (Invited Paper)]
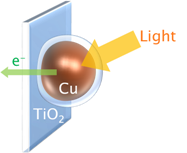
Reversible Drawing of Invisible Images
We have achieved infrared photochromism by using silver nanorods deposited on TiO2. (1) Invisible images that are viewable with an infrared camera can be drawn by infrared light; (2) the invisible image can be overlaid on a visible image drawn by visible light; (3) two different images can be overlaid, and each of them is selectively viewable with an infrared camera with an orthogonally oriented, polarized filter; (4) different images can be erased and drawn repeatedly. The photochromism is based on reversible changes in the nanorod length based on PICS. [Chem Commun, 2012; featured on the cover]

Photocatalytic Remote Oxidation with Visible Light
We found photocatalytic remote oxidation induced by UV light and applied it to photocatalytic lithography. Here we newly found that photocatalytic remote oxidation can also be induced by visible light using photocatalysts such as WO3 modified with platinum nanoparticles. Its mechanism was suggested to be different from that of UV-induced photocatalytic remote oxidation: the visible light-induced photocatalytic remote oxidation may be due to direct oxidation by airborne hydroxyl radical. [J Phys Chem C, 2011]
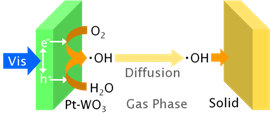
Top Page